Research
The research program of the group is dedicated to unravel mechanisms and processes in the coupling of physical, chemical, and biologic structures on the nanometer scale. This includes excitation and transport in composite structures at surfaces made up of metal nanoparticles, molecule aggregates and proteins. We seek to elucidate the influence of the local environment on the respective processes underlying energy transfer, chemical reactions, and biophysical interactions. The studies require local probing approaches, such as scanning probe microscopy, adapted for the specific system and environment of the process under study.
Scanning Probe and Correlative Microscopy
Scanning probe microscopy methods provide the opportunity to study and modify nanoscopic landscapes down to molecular or atomic scale. Our studies range from wave function mapping with atomic precision at low-temperatures and ultra-high vacuum to transport measurements and manipulation in liquids, with conditions similar to those in living cells. Key issues concern spatial resolution, probing specificity, structuring, and adaptation to special environmental conditions [1{5]. Accessing different information channels from the very same region or structure on the surface enables exploration of complex mechanisms and interplays. This is pursued by the so-called correlative microscopy, which merges morphological, optical, and spectroscopic data, e.g., from scanning probe, photoelectron, and luminescence maps. At nanoscopic dimensions this approach requires proper navigation and setting of landmarks.
Transport of Excitations in Molecular Aggregates
Light-matter interaction can result in a variety of phenomena, such as collective electron oscillations in metals, excitons in molecules, or electron-hole pairs in semiconductors. We are interested in effects arising from the coupling among these phenomena and their interaction with inhomogeneities of the surrounding system. We aim at real-space visualization of exciton transport in molecular aggregates by means of photoemission electron microscopy (PEEM). Metal nanostructures serve as well-defined local excitation sources for excitons in adjoining molecular aggregates. In comparison to fluorescence microscopy, PEEM directly detects the electron occupation rather than de-excitation channels from excited states. This provides additional, complementary information enabling direct comparison of state occupation and de-excitation.
Nanostructuring Molecular Layers
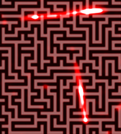
Self-assembly of molecular aggregates is a convenient approach to arrive at nano- or mesoscopic structures. However, control over the aggregation is limited. To address questions how anisotropy, electronic properties, structure motifs, and geometric restrictions affect exciton propagation, creation of well-defined molecular nanostructure landscapes is important. We aim at combining long-range ordering by self-assembly and modification by nanolithography techniques, potentially enabling landscapes for controlled confinement and guidance of charges, light, and excitations. Local excitation can be achieved by plasmonic structures, such as arrays of nanotriangles prepared by nanosphere lithography. An indication of plasmon-exciton coupling is enhanced fluorescence of the molecule aggregate at the sites of plasmonic near fields. Using spatially-resolved fluorescence detection, different local configurations can be compared to each other, enabling identification of active sites and extraction of enhancement factors within a single measurement. Triplet states are particularly promising candidates for long-range exciton mobility beyond the Forster mechanism. Hence, phosphorescence at larger wavelengths, in comparison to shorter-wavelength fluorescence, is suited to select potential molecule species and configurations for exciton transport. Such data serve as a basis for studying the interplay of spatially-dependent excitation, exciton transport, and optical guidance in nano- and microstructured molecule assemblies.
Interaction of Live Cells at Surfaces
Ion flow through nanopipettes represents an extremely gentle type of local interaction suitable for nanoprobing on very soft material surfaces, i.e., on live cells. Unlike atomic force microscopy, in Scanning Ion Conductance Microscopy (SICM) hardly any appreciable forces are applied. This means that the measured nanomorphology and functional maps reflect the undistorted situation of the live cell in the medium. The ion current through the nanopipettes depends on the distance from insulating objects and on the local ion concentration. The spatial resolution is determined by the diameter of the nanopipettes, which are prepared from borosilicate in a laser-based puller. Osteoblasts are seeded and cultured on a surface in a Petri dish. We study morphological changes at migration fronts of osteoblasts on the nanoscale in response to triggers such as geometric, molecular, and electric field landscapes.
Methods
- Scanning Probe Microscopy (SPM)
- Photoemission Electron microscopy (PEEM)
- Scanning Electron Microscopy
- Nanostructuration and -manipulation
Projects
Charge Transfer and Distribution on Surfaces for the Assessment of space-weather induced distortions at technical systems in space (German Aerospace Center Institute for Solar-Terrestrial Physics, DLR)
Charge deposition from solar wind on surfaces of satellites can induce discharge and electronic failure. On oxidic surfaces the lifetime of charges is long and strategies to avoid damage are sought for. Parameters such as charge density, lifetime and mobility of charges on model surfaces are important. To this end we investigate charge distributions from electron beam or plasma deposition under ultrahigh vacuum conditions by multiprobe scanning probe microscopy methods and in situ electron microscopy.
Collaborative Research Centre 1270 'ELAINE': A03 - Material surface charges and their influence on cell physiology and morphology
This Project addresses the relation and mechanisms of interaction between osteoblasts and titanium surfaces, in particular the role of material surface charges on cell behaviour. The project will study how structural, chemical, and electric parameters at the interface to the bio-system and their interplay govern cellular physiology (i.a. calcium signalling) and morphology (i.a. the migration front zone via Scanning Ion Conductance-Microscopy). To this end, methods of surface functionalisation, structuring, nanoprobing, and cell biology will be combined.